LED Characterization
LED Characterization
LED Characterization
LEDs are used today in many applications including lighting of homes, streets, and businesses where they are beginning to supplant more traditional light sources. Despite their advantages, measurement techniques of the power, flux (equivalent to power but measured in units of lumens), and spectrum of LEDs is not very different from that of traditional light sources. The main reason for this is that these sources emit incoherent light, which radiates uniformly in all directions. These sources produce highly divergent light emission. Accurately measuring the power of such sources using a sensor with a fixed aperture size requires sophisticated collection geometries.
Integrating Spheres
A power sensor with a finite aperture, such as one of the photodiodes, is unable to measure the entire beam of a highly-divergent light source. Furthermore, estimating the fraction of power measured through the aperture is not as straightforward as it is for a laser with a well-defined beam size. Instead, a light-collection device known as an integrating sphere can be used in conjunction with a sensitive photodiode for determining the power of a highly-divergent source such as an LED. As shown in Figure 1, an integrating sphere has its inner surface coated with a highly-reflective surface. When a divergent beam hits the walls of the integrating sphere, the light is reflected and scattered many times until the light hitting any place on the walls of the sphere has the same intensity. A sensor placed in the sphere thus gets the same intensity as anywhere else; the power the sensor detects is proportional to the total incident power independent of the beam divergence. To accomplish this, the sensor must be placed so that it only sees scattered light and not the incident beam. Since the integrating sphere divides the beam uniformly in the sphere, a photodiode can measure the fraction of power through its aperture and then determine the total beam power by knowing the ratio of the area of the aperture to that of the entire sphere. The integrating sphere also lowers the power entering the photodiode, so one can measure much higher input beam powers.
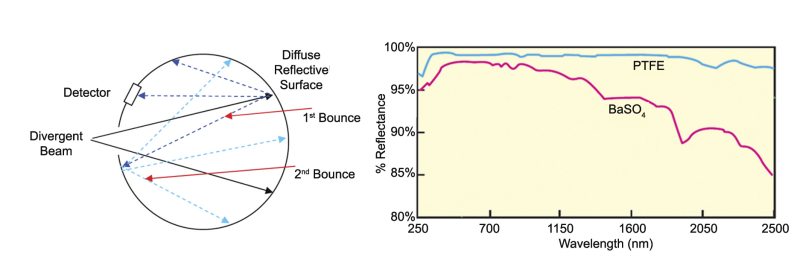
Figure 2 shows our integrating spheres which are designed to be configured in various ways for many applications. With an extensive line of sphere accessories available, a single sphere can perform various integrating sphere tasks such as uniform illumination, light measurement, and reflectance measurement with reasonable accuracy. The spheres have a highly-reflective diffuse white coating (see Figure 1) for high efficiency and readings that are independent of beam size, position, and divergence. The spheres can accommodate light emission in the UV, VIS, and NIR spectral regions while handling input powers up to 30 W. The spheres also come in different sizes since the sphere must scale in size with the input beam aperture to ensure uniform scattering. The larger diameter spheres can be used with either divergent beams, e.g., LEDs, laser diodes, fiber output, or collimated beams from lasers (see Figure 2). This is accomplished by setting the optical geometry such that the input beam never directly hits the sensor and the sensor only sees rays reflected from the wall, as illustrated rightmost in Figure 2. To maintain accuracy, annual integrating sphere sensor calibration is recommended. Finally, any number of the thermopile or photodiode sensors could be suitable choices for LED measurement in combination with an integrating sphere.
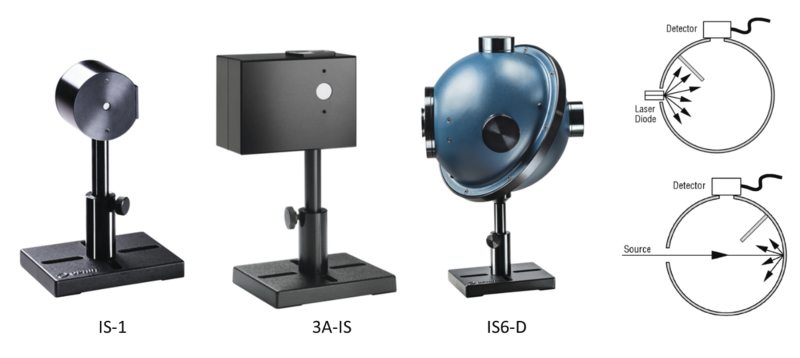
FluxGage™
The integrating sphere is the standard instrument for measuring power, flux, and color of LED lights. However, the integrating sphere must be at least three times the size of the device under test (DUT). This ensures that the light from the DUT is uniformly scattered within the sphere prior to reaching the sensor and enables an accurate power estimate. For large LED luminaires or lamp assemblies that can approach 2-3 feet, this requires integrating spheres with diameters of 6-10 feet for proper characterization. Additionally, the integrating sphere must be recalibrated for each use to account for the absorption of the DUT itself. MKS developed the FluxGage™ measuring system (see Figure 3) to address these issues using a unique approach: employ detection surfaces which absorb and detect light. By eliminating the need for diffuse reflections, the characterization device can be the size of the DUT, e.g. rectangular, and be calibrated only once. The detection surfaces should be able to efficiently detect light, be independent of incidence angle, and absorb all the light with very little reflection. The FluxGage system's detection surface (see Figure 3) is made of solar panels to detect light, a sheet diffuser to make the panels insensitive to angle, and a dense array of pinholes printed with black ink over the diffuser. This arrangement ensures all three requirements are met.
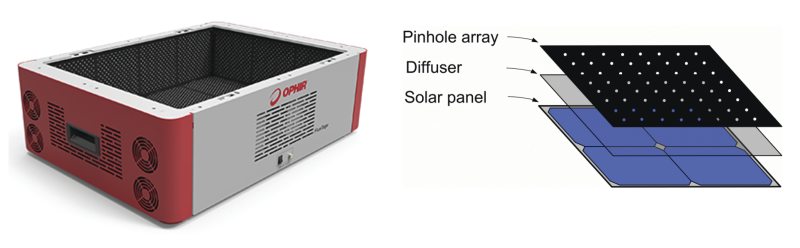
The FluxGage system is therefore a compact measuring system for LED Luminaires. The system measures total flux or power using solar panels while an onboard spectrometer and fast photodiode complete the system and provide color and flicker (oscillation in power output) measurements. The benefits of using the FluxGage system are small size, robustness, production readiness, ease of use, and less frequent calibration. Increased demand for LED luminaires requires manufacturing operations to adapt to improved quality testing methods that can provide quality measurements while not slowing down the production lines. The value of using the FluxGage system is its ability to take key measurements quickly, but also to allow the manufacturing operation to test the entire luminaire as a fully performing, operational lamp.